Written by Srikanth Gorthi, Lav Khot, Gwen-Alyn Hoheisel, Washington State University, 01 February 2024.
Air temperature is used to predict lethal temperatures for sweet cherry buds, but it can differ from the actual bud tissue temperature. We compared different types of sensors to quantify bud tissue temperature in 2022 and 2023 spring seasons. A significant difference between air and bud tissue temperature was observed during spring frost nights. Bud tissue temperature dropped rapidly below 32°F compared to air temperature. The relationship between air and bud tissue temperature could be used to better predict lethal temperatures.
Spring Frost Management
Sweet cherry production is challenged by frost damage particularly in the early spring. In 2022-23 season, the net fruit yield across Washington State was reduced by 21% due to cold injury in the late winter and early spring seasons (USDA NASS, 2022). As buds develop in the early spring, they deacclimate to cold temperatures. Occasional warmer temperatures in early spring can expedite the onset of bud break making them more susceptible to frost damage from subsequent colder temperatures. Therefore, knowing the critical temperature in which sweet cherry buds are killed is vital for timely active frost mitigation like heaters and wind machines. Cold hardiness models use air temperature as a predictor to forecast the cultivar-specific critical temperatures. However, crop tissues exhibit a unique heat-holding capacity that can affect the tissue temperature during clear sky nights or direct exposure to daylight (Millar, 1972; Landsberg et al., 1974; Snyder et al., 2005). Thus, using air temperature may lead to inaccuracies in predicting critical temperatures and resulting in premature or delayed actuation of active frost mitigation. We conducted a series of experiments to better understand the relationship between air and bud tissue temperature as well as sensors to measure them.
Sensing Bud Tissue Temperature
In the 2022 and 2023 spring seasons in three sweet cherry (Prunus avium cv. Chelan) orchard blocks in central WA, we tested a direct contact sensor (thermocouple) and a non-contact sensor (radiative frost) against the control of a standard air temperature sensor. Precision type-E thermocouples (Omega Engineering, Norwalk, CT, USA; probe diameter: 0.005 inch) were inserted into the dormant flower buds for direct bud tissue temperature (Tc) measurement. They were pushed gently inside the buds until the probe tip reached about 1/64 inch (0.2 mm) (Figure 1a). Similarly, thermocouples were inserted into the flowers as shown in Figure 1b.
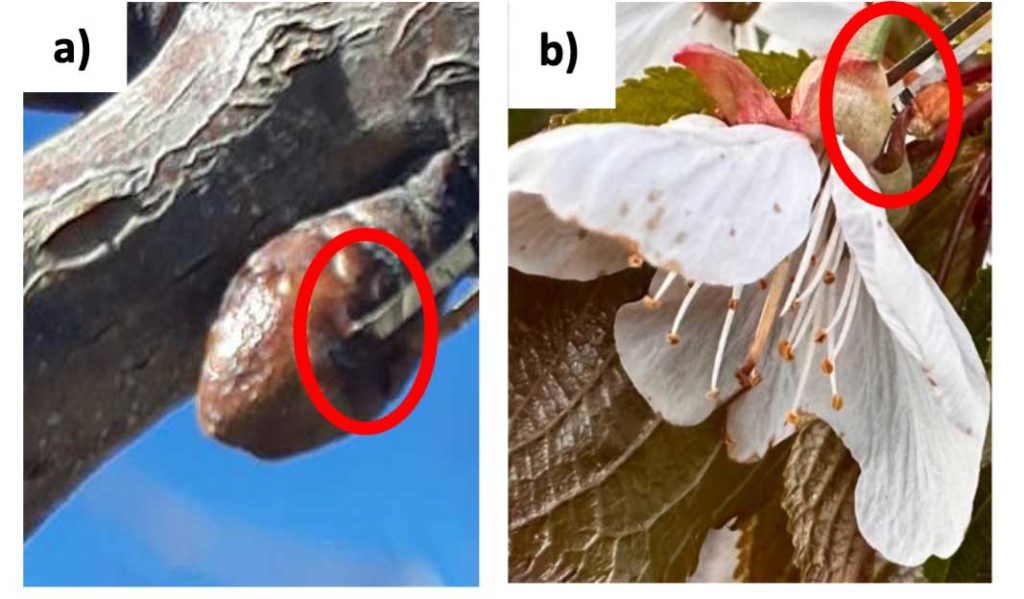
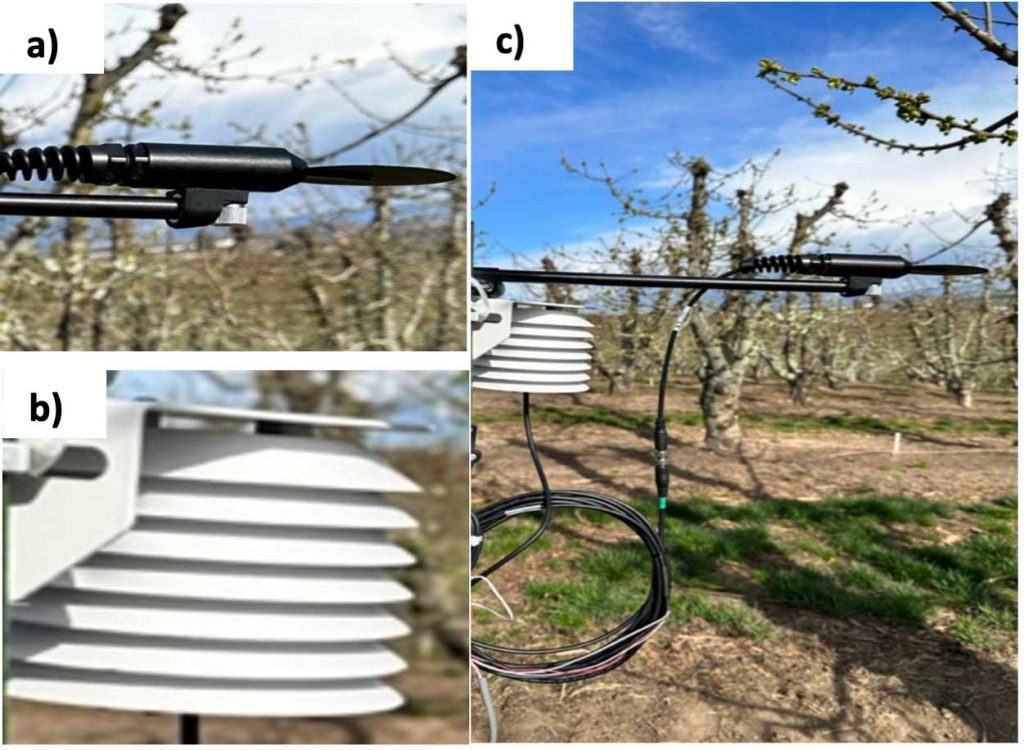
For indirect bud tissue temperature measurements, a radiative frost sensor (SF-421, Apogee Instruments, Inc. Logan, Utah, USA; range: –58 to 160 °F) was evaluated. This sensor consists of a precision thermistor attached to a 2.3-inch disk (Figure 2a). Per manufacturers’ document, it is designed to simulate leaf temperature (Trf) during spring frost events. The thermistor is reported to have a similar heat capacity as the plant leaves. This sensor was deployed along with an aspirated air temperature (Ta) probe (ATMOS-14, Meter Group, Inc. Pullman, WA, USA; Ta range: –40 to 180 °F) at five feet above ground level (Figure 2c). The latter quantified in-orchard air temperature and relative humidity.
Bud Tissue and Air Temperature Offsets
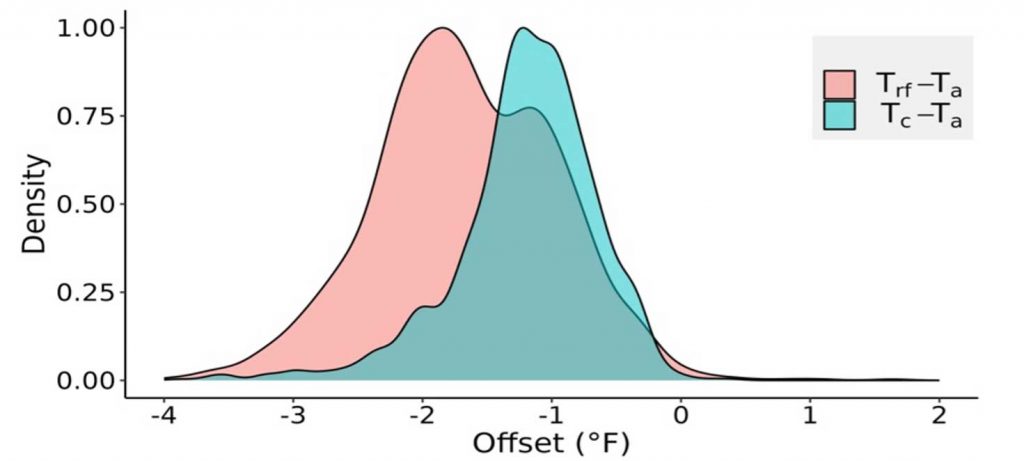
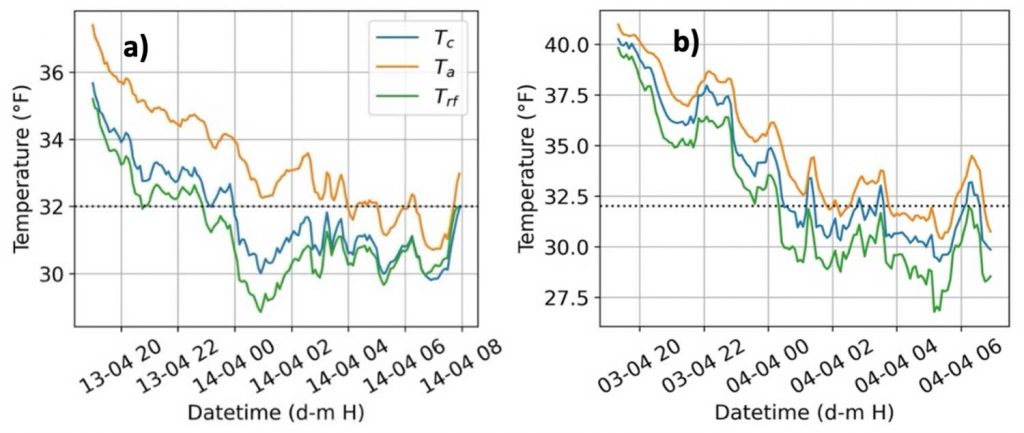
Statistical tests showed a significant difference among the mean nighttime (1800 – 0700 hours) temperatures (Ta, Trf, and Tc) indicating that both air temperature and radiative frost sensors record different temperatures than the actual bud tissue temperature. However, since air temperature is the traditional measurement for frost management, we calculated the temperature difference, or offset, between the bud tissue temperature sensors from the air temperature (Figure 3). The offset temperatures for both sensors (Trf and Tc) varied from Ta by –4 to 0.2 °F. The mean offset for the direct (Tc) sensor was –1.35 °F, but much higher at –3.15 °F for the indirect (Trf) sensor. The indirect radiative frost sensor recorded lower bud tissue temperature due to the large sensing surface area compared to direct thermocouple measurement. A slight positive offset (Figure 3) for bud tissue temperature can be attributed to bud tissue temperature increase during the frost mitigation. Using only air temperature (Ta) for actuating frost mitigation could be problematic as i) Ta was consistently higher than bud tissue temperature (Tc), and ii) the bud tissue and radiative frost sensors recorded below freezing temperatures (32oF) at an earlier time than Ta (Figure 4). The offsets or temperature differences for Trf and Tc with Ta were more variable during spring frost events, which may also be influenced by other weather parameters like relative humidity, wind speed, and cloud cover. Due to the observed offsets, cold hardiness models estimating lethal temperatures using only air temperature may result in mitigation errors as air and bud tissue temperature are significantly different. More research is warranted to estimate the errors of cold hardiness model predictions that use air temperature instead of bud tissue temperature.
Contact
Srikanth Gorthi
Washington State University
Graduate Student
Lav Khot
Washington State University
AgWeatherNet Director
Gwen-Alyn Hoheisel
Washington State University
Regional Extension Specialist in Tree Fruit, Grape, and Berry
Affiliate in Center for Precision and Automated Agricultural Systems
Acknowledgments
This study was supported in part by NSF/USDA NIFA Cyber-Physical Systems (Award No: 2021-67021-34336), AgAID Institute, the Washington State Department of Agriculture (WSDA) Specialty Crop Block Grant, and USDA NIFA 0745 projects. The authors want to acknowledge Ms. Karisma Yumnam, Dr. Basavaraj Amogi, and Mr. Laurent Leonard of Washington State University for their assistance in data collection and analysis. The authors also thank grower cooperators (Olsen Farms, Lighthouse Farms, and Hayden Farms) for supporting this research.
Additional Reading
Landsberg, J. J., Butler, D. R., & Thorpe, M. R. (1974). Apple bud and blossom temperatures. Journal of Horticultural Science, 49(3), 227–239.
Millar, A. A. (1972). Thermal Regime of Grapevines. American Journal of Enology and Viticulture, 23(4), 173–176.
Snyder, R. L., Melo-Abreu, J. P. de, & Matulich, S. (2005). Frost protection: Fundamentals, practice and economics (Vol. 2). Food and Agriculture Organization of the United Nations.
USDA NASS. (2022). Cherry Production [Press Release]. United States Department of Agriculture.
Fruit Matters articles may only be republished with prior author permission © Washington State University. Reprint articles with permission must include: Originally published by Washington State Tree Fruit Extension Fruit Matters at treefruit.wsu.edu and a link to the original article.