Written by Marcella Galeni, Washington State University–Irrigated Agriculture Research and Extension Center and Carolina Torres, Washington State University–Tree Fruit Research and Extension Center, Wenatchee.
Organic apple production in Washington represented 13% of the total harvest of apples in 2022 (Fruit Growers News, 2022). One of the biggest challenges for success and profitability is being able to store the fruit for more than eight months. In the absence diphenylamine (DPA) and 1-methylcyclopropene (1-MCP) to prevent superficial scald and slow down ripening, the use of controlled atmosphere (dynamic, low oxygen, and ultra-low oxygen) is the only chemical-free alternative to achieve it. Below we present a brief description of storage systems, including the use of low-pressure storage, which could become commercial in the future, as well as some results from a project conducted in ‘Honeycrisp’ and ‘Fuji’ apples, both of which are second and third in volume in Washington (Granatstein and Kirby, 2021).
Controlled Atmosphere Storage
Controlled atmosphere (CA) storage is a storage system widely used for storage of apples. In apples, 1%-3% O2 and 0.5%-2.5% carbon dioxide levels are commonly used, allowing long-term preservation through the decrease of ethylene production, slowing down ripening and senescence processes (Thompson et al., 2010). This system can also induce the development of physiological disorders associated with carbon dioxide toxicity (Figure 1) and internal browning (Prange and DeLong, 2006).
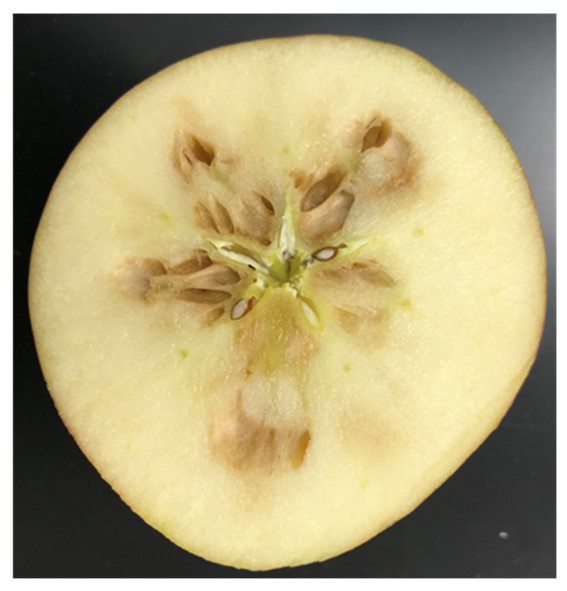
The recommended levels of oxygen and carbon dioxide in CA storage of apples can fluctuate according to where the fruit was cultivated showing differences among growing areas. In Washington State, ‘Honeycrisp’ is generally stored at 2%-4% oxygen and 0.8%-1% carbon dioxide at 37°F and ‘Fuji’ at 1%-2% oxygen and less than 1.0% carbon dioxide at 33°F commercially.
DYNAMIC CONTROLLED ATMOSPHERE STORAGE
Dynamic controlled atmosphere storage refers to the CA system where the oxygen level in managed dynamically throughout the storage season, according to the low oxygen level (LOL), which is the level of oxygen in storage before fermentation occurs. LOL can be determined by different methods directly or indirectly measuring the anaerobic compensation point (ACP) (Mditshwa et al., 2018). The anaerobic compensation point (ACP) is the level of oxygen where the metabolism of the fruit reaches a level that leads to fermentation. The commercial systems available to do DCA are chlorophyll fluorescence, respiratory quotient and ethanol concentration (Mditshwa et al., 2018). In our study, initial low oxygen stress (10 days prior CA conditions) and respiratory quotient storage systems decreased the incidence of soft scald after nine months of storage in comparison to standard CA storage, but the results varied amount seasons (Figure 2).
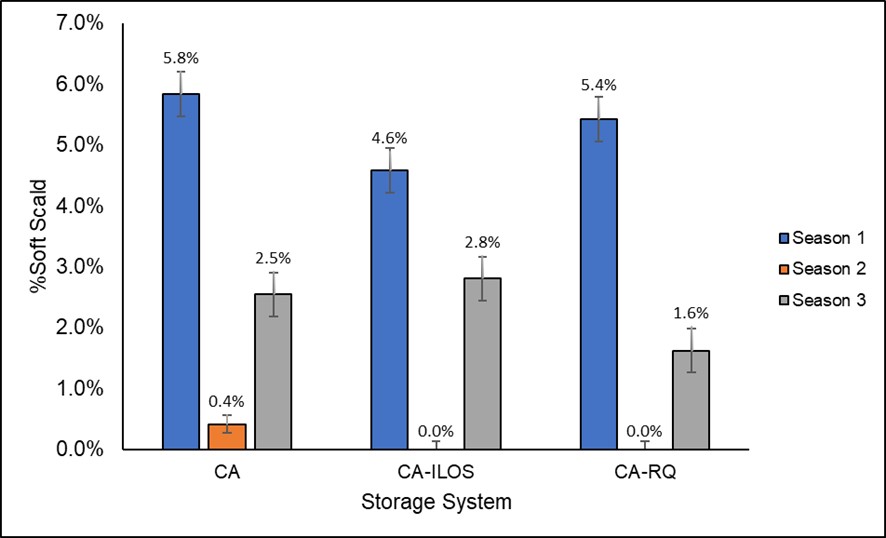
Chlorophyll Fluorescence
The HarvestWatch® using a FIRM sensor measures the chlorophyll fluorescence (CF) on the fruit surface non-destructively during cold storage. This parameter correlates to low oxygen and high carbon dioxide stress that fruit is experiencing during this time (Prange et al., 2003) (Figure 3). The chlorophyll fluorescence (Fα) will increase when fermentation occurs indicating the LOL was detected. The increase in chlorophyll fluorescence allows the operators to monitor the fruit and increase the oxygen level above the LOL to prevent fermentation (Zanella et al., 2005).
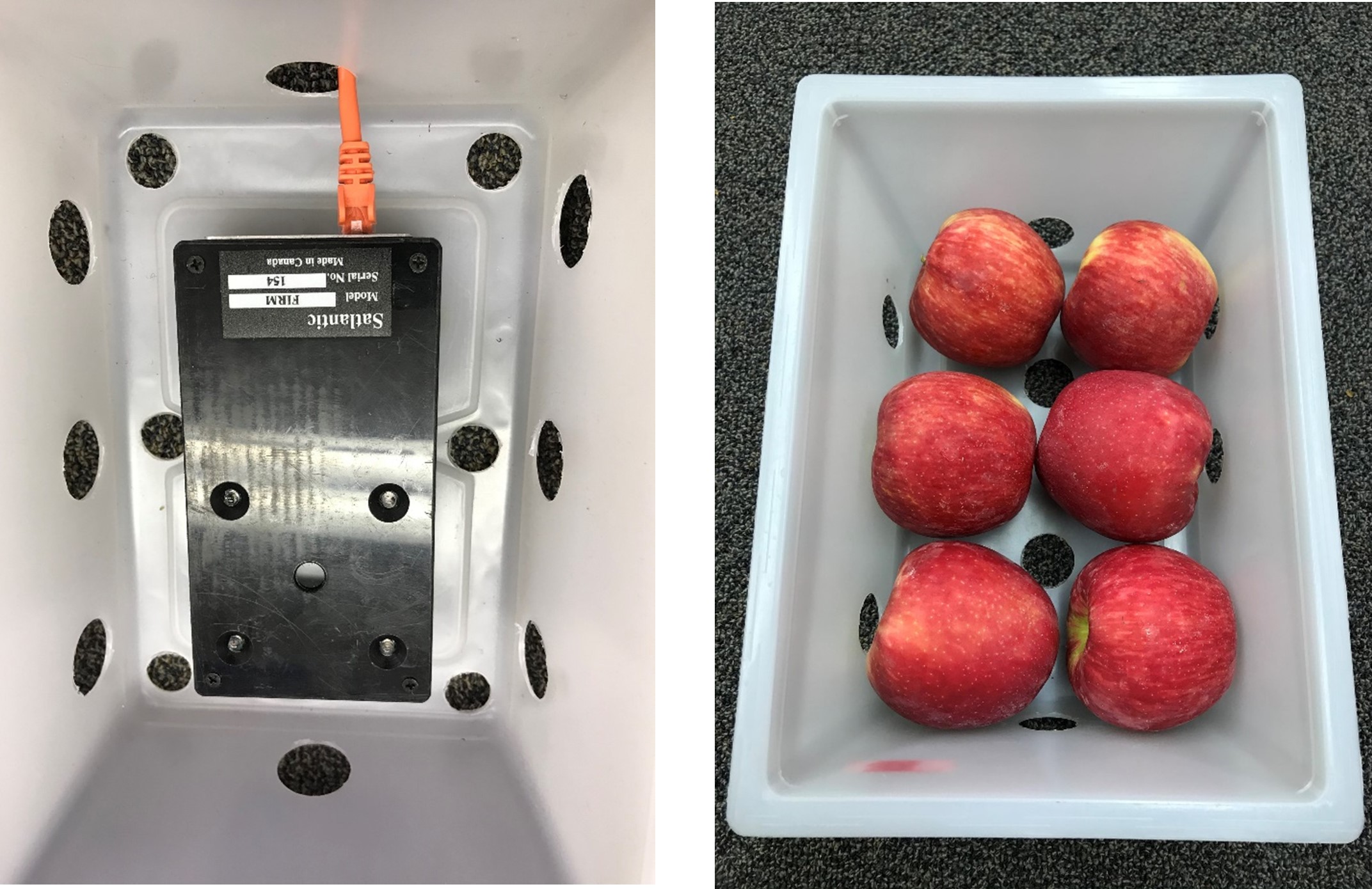
Respiratory Quotient
The respiratory quotient (RQ) systems monitor the ACP through the ratio of carbon dioxide produced and oxygen consumed by the stored produce (Mditshwa et al., 2018). A RQ value above 1.0 indicates a transition from aerobic to anaerobic or fermentative metabolism (Weber et al., 2020). SafePod (Storage Control Systems Inc., Sparta, MI, USA) is a RQ system commercially available (Figure 4).
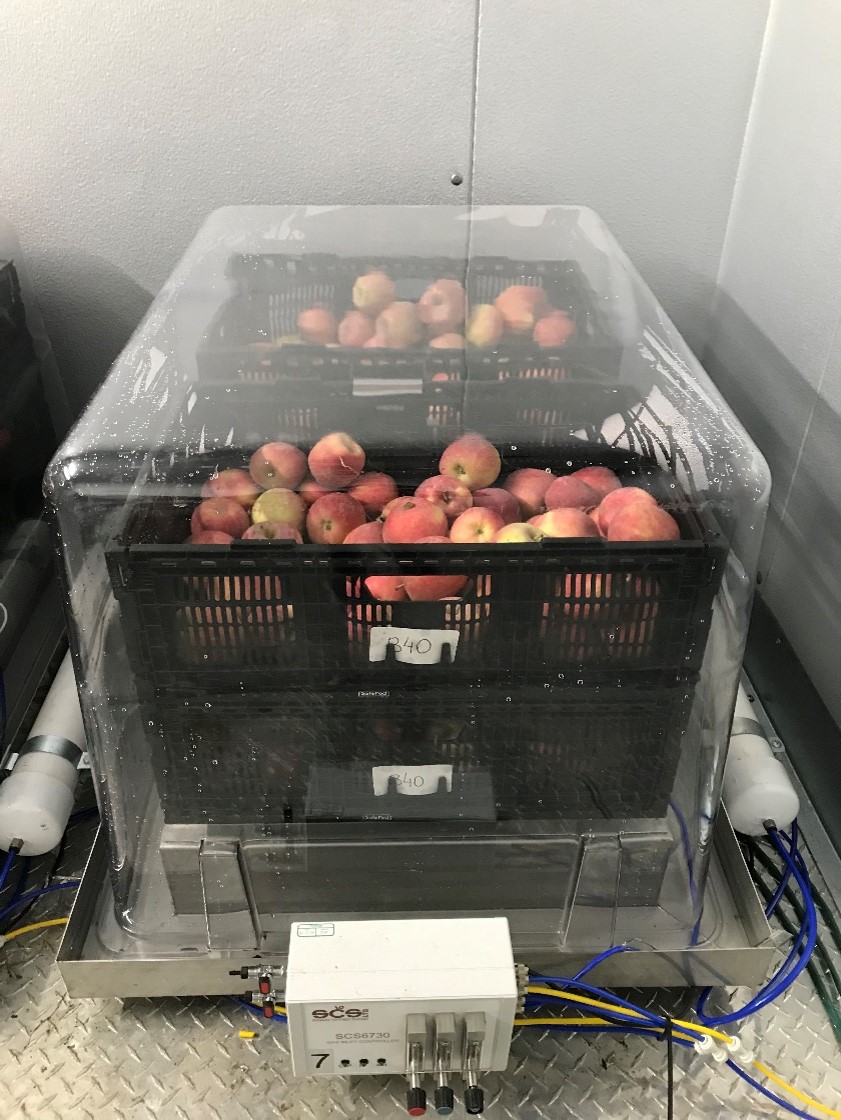
Ethanol Concentration
Another method to monitor anerobic respiration when low oxygen levels are used in storage, is through the measurement of ethanol concentration. Initial low oxygen stress (ILOS) prior to the establishment of CA storage has shown benefits by reducing superficial scald and firmness loss in apples (Wang and Dilley, 2001; Zanella, 2003). This system consists of lowering oxygen levels immediately after harvest for 1-2 weeks to induce ethanol accumulation in the fruit Wright et al., 2015). Performing ILOS before CA storage at 1kPa O2 can be an alternative for DPA use against superficial scald for ‘Granny Smith’ apples (Zanella, 2003).
Hypobaric or Low-pressure Storage
Hypobaric or low-pressure storage is a system that stores horticultural commodities by ventilating air at less than atmospheric pressure, extending the useful life of perishable commodities past that attained by CA storage at the same oxygen levels (Wang and Dilley, 2000). Hypobaric storage works by not only decreasing ethylene, but also removes other gaseous such as carbon dioxide within the stored produce, thereby delaying the ripening process (Burg, 2004). Hypobaric storage involves the use of a vacuum pump, which constantly removes air from the store to retain the desired oxygen level since the stored commodity is continuously respiring (Thompson, 2016). However, the removal of air from the storage reduces the respiration rate and leads to rapid water loss by the commodity; therefore, the commodity needs to be maintained at a humidity closest to saturation (100% RH) to limit the loss of water in storage. In our study, respiration rate is lower in low pressure storage than in CA storage for both seasons (Figure 5). The low-pressure storage chambers were provided by Ripelocker, LLC. The system dynamically manage pressure, oxygen and carbon dioxide inside the chambers. The system is commercially available for blueberries, cut flowers, and hops, but further research needs to be performed in Apples. Our objective in the study was to modulate chilling injury disorders such as soft scald and soggy breakdown in ‘Honeycrisp’ apples (Figure 6). For this reason, we chose to store ‘Honeycrisp’ apples under 33°F instead of 37°F, which is the recommended temperature for the cultivar. Prolong shelf-life after storage as well as delay softening, decrease decay and control of physiological disorders are some of the advantages of storing apples stored under hypobaric conditions (Thompson, 2016). While hypobaric storage has many advantages over CA storage, the system is not widely used in the United States because of cost associated with the technology (Paskus et al., 2021). No commercial hypobaric or low-pressure storage is currently available for growers and packers.
Figure 5. Respiration rate of ‘Honeycrisp’ apples after nine months of CA storage plus four weeks in Air storage plus seven days at room temperature. Abbreviations: CA (controlled atmosphere storage), LP (low-pressure storage). The data into graph is displayed as average and standard error.
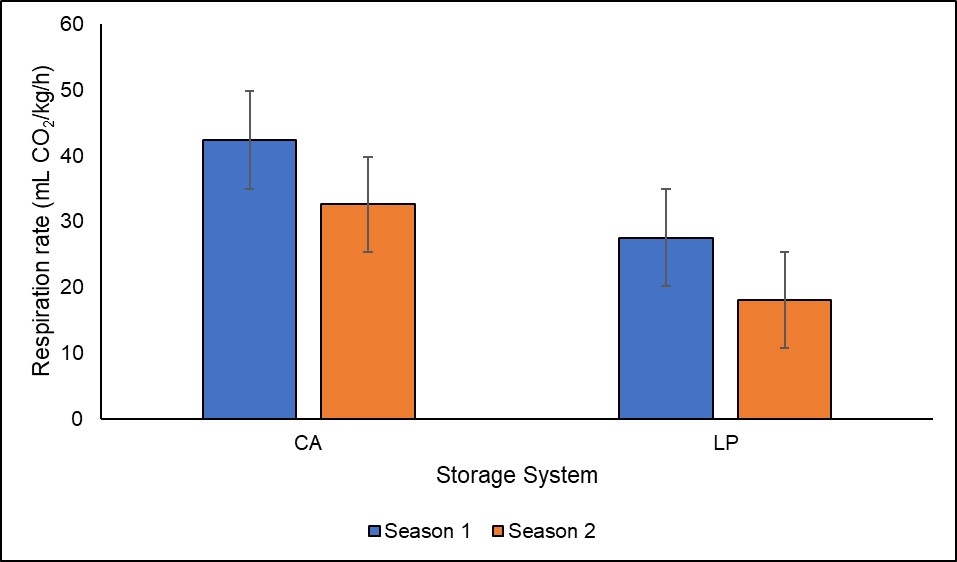
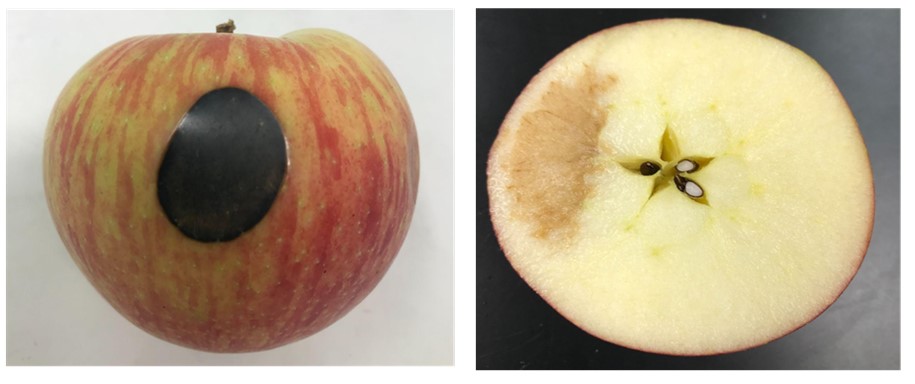
References
Burg, S.P., 2004. Origins of the LP concept, In: Postharvest Physiology and Hypobaric Storage of Fresh Produce. CABI Wallingford, Oxon, UK, Cambridge, MA, USA, pp. 9–17. https://doi.org/10.1079/9780851998015.0000
Granatstein, D., Kirby, E., 2021. Recent Trends in Certified Organic Tree Fruit. Wenatchee, WA. https://s3-us-west-2.amazonaws.com/tfrec.cahnrs.wsu.edu/wp-content/uploads/sites/9/2021/03/WA_OrgTreeFruit_ann_rev_2020.pdf
Fruit Growers News, 2022. Washington’s fresh apple crop is smaller, but growers optimistic. https://fruitgrowersnews.com/news/washingtons-fresh-apple-crop-is-smaller-but-growers-optimistic/
Mditshwa, A., Fawole, O.A., Opara, U.L., 2018. Recent developments on dynamic controlled atmosphere storage of apples—A review. Food Packaging and Shelf Life 16, 59–68. https://doi.org/10.1016/j.fpsl.2018.01.011
Paskus, B., Abeli, P., Beaudry, R., 2021., Hypobaric Storage of Representative Root, Leaf, Fruit, and Flower Tissues: Comparisons to Storage at Atmospheric Pressure and Normoxia. HortScience 56, 780–786. https://doi.org/10.21273/HORTSCI15786-21
Prange, R.K., DeLong, J.M., 2006. Controlled-atmosphere related disorders of fruits and vegetables. Stewart Postharvest Review. 5(7), 1-10.
Prange, R.K., DeLong, J.M., Harrison, P.A., Leyte, J.C., McLean, S.D., 2003. Oxygen concentration affects chlorophyll fluorescence in chlorophyll-containing fruit and vegetables. J. Am. Soc. Hortic. Sci. 128, 603–607. https://doi.org/10.21273/jashs.128.4.0603
Thompson, A.K., 2010. CA Technology, In: Controlled Atmosphere Storage of Fruits and Vegetables. CABI, Wallingford, UK. pp. 26-44. https://doi.org/10.1079/9781845936464.0000
Thompson, A.K., 2016. Hypobaric Storage, in: Fruit and Vegetable Storage: Hypobaric, Hyperbaric and Controlled Atmosphere. Springer, Huddersfield, UK, pp. 37–92. https://doi.org/10.1007/978-3-319-23591-2
Wang, Z., Dilley, D.R., 2000. Initial low oxygen stress controls superficial scald of apples. Postharvest Biol. Technol. 18, 201–213. https://doi.org/10.1016/S0925-5214(00)00067-3
Wang, Z., Dilley, D.R., 2001. Initial Low Oxygen Stress (ILOS) Controls Scald of Apples Without Using Postharvest Chemical Treatments. Acta Horitculture 21, 261–266.
Weber, A., Neuwald, D.A., Kittemann, D., Thewes, F.R., Both, V., Brackmann, A., 2020. Influence of respiratory quotient dynamic controlled atmosphere (DCA – RQ) and ethanol application on softening of Braeburn apples. Food Chem. 303, 125346. https://doi.org/10.1016/j.foodchem.2019.125346
Wright, A.H., Delong, J.M., Arul, J., Prange, R.K., 2015. The trend toward lower oxygen levels during apple (Malus × domestica Borkh) storage – A review. J. Hortic. Sci. Biotechnol. 90, 1–13. https://doi.org/10.1080/14620316.2015.11513146
Zanella, A., 2003. Control of apple superficial scald and ripening—a comparison between 1-methylcyclopropene and diphenylamine postharvest treatments, initial low oxygen stress and ultra low oxygen storage. Postharvest Biology and Technology, 27(1), 69–78. https://doi.org/10.1016/S0925-5214(02)00187-4
Zanella, A., Cazzanelli, P., Panarese, A., Coser, M., Cecchinel, M., Rossi, O., 2005. Fruit fluorescence response to low oxygen stress: Modern storage technologies compared to 1-MCP treatment of apple. Acta Hortic. 682, 1535–1542. https://doi.org/10.17660/ActaHortic.2005.682.204
Contact
Marcella Galeni
Scientific Assistant, Stone Fruit Breeding & Genetics
WSU Irrigated Agriculture Research and Extension Center
Prosser, WA
phone: 509-786-9306
email: m.galenisoaresrafae@wsu.edu
Carolina Torres
Endowed Chair Postharvest Systems, Horticulture
WSU Tree Fruit Research & Extension Center
Wenatchee, WA
phone: 509-293-8808
email: ctorres@wsu.edu
Funding and acknowledgements
Washington Tree Fruit Research Commission
Project Title: Postharvest system optimization for organic apple storage (2019-2021)